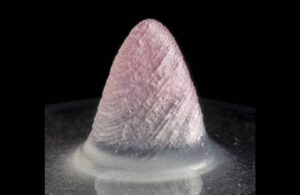
The tissue-engineered 3D ventricle model. Image credit: Harvard SEAS
Researchers have found a way to use fiber-infused ink to 3D-print a functional heart ventricle that mimics the beating of a human heart.
The team included researchers from Harvard John A. Paulson School of Engineering and Applied Sciences (SEAS) and the Wyss Institute for Biologically Inspired Engineering at Harvard University. They reported on their new hydrogel ink infused with gelatin fibers in a paper published in Nature Materials.
This fiber-infused gel (FIG) ink allows heart muscle cells printed in the shape of a ventricle to align, beating in coordination like a human heart chamber.
“People have been trying to replicate organ structures and functions to test drug safety and efficacy as a way of predicting what might happen in the clinical setting,” said Suji Choi, research associate at SEAS and first author on the paper.
Choi explained that FIG ink can flow through the printing nozzle and maintains its 3D shape. That makes it possible to print a ventricle-like structure and other complex 3D shapes without using extra support materials or scaffolds.
Using a previously published rotary jet spinning technique, Choi fabricated microfiber materials in a manner similar to the spinning of cotton candy, creating the ink. Co-author Luke MacQueen, a postdoctoral researcher and Wyss Lumineer, proposed the jet-spinning idea. With Choi, they developed this technique in the lab of Kevin Parker.
“When Luke developed this concept, the vision was to broaden the range of spatial scales that could be printed with 3D printers by dropping the bottom out of the lower limits, taking it down to the nanometer scale,” Parker said. “The advantage of producing the fibers with rotary jet spinning rather than electrospinning” — a more conventional method for generating ultrathin fibers — “is that we can use proteins that would otherwise be degraded by the electrical fields in electrospinning.”
Using the jet-spinning technique to spin gelatin fibers, Choi produced a sheet of material similar in appearance to cotton. Then, she used sonification-sound waves, breaking the sheet into fibers about 80 to 100 micrometers long and about 5 to 10 micrometers in diameter. The next step was to disperse the fibers into the hydrogel ink. According to Wyss, Choi found the most difficulty troubleshooting the ratio between fibers and hydrogel. She wanted to maintain fiber alignment and the overall integrity of the 3D-printed structure.
Choi printed 2D and 3D structures with the ink and saw cardiomyocytes (the cells responsible for heart muscle contractions by transmitting coordinated electrical signals) line up in tandem with the direction of the fibers inside the ink. With control of the printing direction, Choi controlled how the heart muscles aligned. In applying electrical stimulation to 3D-printed structures made with FIG ink, she saw a coordinated wave of contractions aligned with the direction of the fibers.
“It was very exciting to see the chamber actually pumping in a similar way to how real heart ventricles pump,” Choi said.
Choi went on to experiment with more printing directions and ink formulas. She determined her ability to generate stronger contractions with ventricle-like shapes. The researchers believe they can use the technique for heart valves, dual-chambered miniature hearts and more.
“FIGs are but one tool we have developed for additive manufacturing,” Parker said. “We have other methods in development as we continue our quest to build human tissues for regenerative therapeutics. The goal is not to be tool driven — we are tool agnostic in our search for a better way to build biology.”
Previously: Researchers develop implantable heart valve that grows with a child